By Michael D. Smart, ‘97
BOOM! BOOM! BOOM!
A series of explosions flashed across the tennis-court-sized pit, sending dirt and water high into the air. It seemed like all of Treasure Island heaved a few feet higher above the lapping waters of San Francisco Bay.
BYU‘s Kyle M. Rollins rushed to the edge of the chest-deep hole, months of meticulous preparation motivating his anticipation to see if the experiment had worked. Once considered a long shot, the study was now an eminently feasible attempt to simulate an earthquake.
Building on a tradition established by Rollins’ BYU colleague T. Leslie Youd, the effort was another in a series of BYU studies of liquefaction, a mysterious geological phenomenon catalyzed by quakes.
First identified in 1964, liquefaction occurs in sandy areas, like those along sea coasts or even rivers and lakes, and anywhere else with loose soil and a high water table. When an earthquake strikes, it rattles grains of sand and gravel against each other. This action rearranges the tiny open spaces between them and causes settling like that which occurs when someone shakes a bucket full of rocks. At the same time, the quake pushes ground water upward until the water pressure exceeds the weight of the sand and soil above it. Then the water pushes the grains of sand apart, turning once-stable soil into quicksand and potentially undermining the foundations of buildings, bridges, and utility poles.
But thanks in large part to Youd and Rollins, buildings in those areas might tilt but not collapse, bridges over those waterways might bend but not break, and people in those cities might panic but not die. Men of starkly different personalities, the two professors of civil engineering have arrived at their international stature within liquefaction research by approaching the subject from different vantage points and working surprisingly little together.
Youd, a gregarious and self-assured storyteller, discovered a form of liquefaction that is particularly deadly and developed a method of predicting its occurrence. Throughout his career, he has traveled the world to study some of the world’s most severe earthquakes, most recently to Turkey in August 1999.
Rollins, whose understated manner belies his successes, focuses on man-made structures and how they react when the surrounding soil liquefies. His ingenious methods of developing and testing foundations strong enough to withstand liquefaction involve a mix of high explosives, a rocket-powered sled, and a multiton weight dropping from the sky.
“I find problems; Kyle solves them,” says Youd, summing up their complementary roles. Indeed, Rollins might never have found himself peering into that sandy crater on Treasure Island without the work done by Youd during the previous three decades.
The Earthquake Chaser
Youd, whose career is twice as long as that of his younger counterpart, began his march to worldwide liquefaction prominence when he was a wet-behind-the-ears officer of the U.S. Geological Survey (USGS) in 1971. Three years out of graduate school, he stepped off a plane onto the tarmac at the Burbank airport and was hustled into a helicopter, its rotors already whirring. Youd and his partner had been dispatched from the USGS offices in Menlo Park, Calif., to observe firsthand the effects of the earthquake that had struck San Fernando only hours earlier. It would be the first of many occasions when Youd would put his desk job on hold to chase an earthquake.
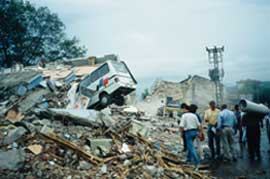
A van trapped in Turkish rubble is a solemn reminder of the thousands of lives taken by buildings that crumbled in the earthquake. Youd’s team examined the ability of structures to withstand a large earthquake. The good news: Structures built to code did well. The bad news: Many buildings were not up to code.
He and fellow researchers congregated at a battered juvenile detention facility, where no one could explain what caused the entire plot of land surrounding the building to slide 6 feet toward a nearby lake. Youd took measurements and asserted that the surface of the ground had floated atop a layer of liquefied soil. A combination of an ever-so-slight slope and the inertial forces of the quake had moved the surface. In Youd’s initial report he called the newly discovered phenomenon a “landslide.” Later a colleague renamed it “lateral spread,” a term which pervades earthquake engineering literature today.
After his successful discovery of lateral spread in San Fernando, Youd surmised that it probably wasn’t the first time the earth had behaved that way.
“We spent hours and hours reading all we could about the 1906 San Francisco earthquake and reinterpreting and identifying many areas of lateral spread,” he explains. It turned out that lateral spread caused the failure of many of the city’s water pipes, which severely hampered fire-fighting efforts. The fire caused 85 percent of the damage wrought by the infamous quake.
With a major discovery under his belt, Youd accepted a professorship at BYU in 1984 and further explored when and where lateral spread might strike.
“If you have buildings on top of it, lateral spread will literally tear them apart,” he says. “A typical well-built foundation might take 2 to 3 feet of displacement (movement) without failing. So engineers want to know how much lateral spread might occur—and how far it might displace the soil—so they can build foundations that will survive it.”
To that end Youd and his then-graduate student Steve Bartlett developed a reliable way to predict how far the ground would move in the event of lateral spread. Now known as the Bartlett/Youd Equation, the method they published in 1992 is used routinely throughout the Americas to predict the degree of displacement engineers must prepare for when building in areas prone to the lateral spread type of liquefaction.
“He is one of the top, if not the top, liquefaction experts in the world,” says Lee Siegel, who was one of the Associated Press’ five science writers before spending seven years as science editor of the Salt Lake Tribune.“It seems every time there is a major earthquake, it’s not long before I hear Les Youd is headed there.”
Since his first helicopter-aided visit to the San Fernando quake site, Youd has continued to participate in what earthquake engineers call (with a dash of military sobriety), “earthquake reconnaissance missions.” Sometimes it seems Youd views them with more of a hunter-chasing-prey aura like that portrayed in the movie Twister than the detached precision of a military mission. He refers to one quake as “a liquefaction delight,” sounding more like he’s talking about a new treat at Dairy Queen than a ruptured fault. But the veteran of 20 earthquake reconnaissance missions is also a grandfather of 11, and the human cost of the disasters he studies is not lost on him. Most poignant are his observations from the August 1999 quake in Turkey.
Youd was appointed by Oakland-based Earthquake Engineering Research Institute (EERI) to lead a team of researchers into the quake-ravaged land days after a 7.5-magnitude quake leveled apartment buildings and killed more than 17,000 people. This quake was of particular interest to the American engineers because Turkey has modern building codes similar to those in the United States. They wanted to see how American standards would hold up against such a powerful temblor.
But when the team arrived in rented jeeps at the city of Golcuk, research took a back seat to pathos.
“We drove slowly past debris on the street, collapsed buildings, search and rescue going on—it’s depressing, disturbing,” Youd explains slowly, switching to the present tense as though he’s reliving the scene. “Lines of people, predominantly women wearing their traditional black Muslim head coverings, intently watching what’s going on. You know they’ve got loved ones in that rubble. They’ve just got the most horrid, somber faces. Chances are almost nil that their loved ones are coming out alive.
“That’s very disturbing to see,” he repeats, still visibly bothered by the images in his mind. “Two-thirds of the buildings in a given area were down. That’s hundreds of buildings. Responders were overwhelmed.”
Youd’s team concluded that the building codes had worked—structures that were up to code held firmly enough to prevent the loss of life. But tragically, contractors skirted or outright ignored the codes on countless buildings, many of them multiunit apartment complexes.
In addition to blaming the engineers who designed the substandard buildings and the contractors who built them, Youd fingers the Turkish government for failing to enforce the codes and developers for encouraging contractors to slash costs.
“If those buildings had been built to code, the loss of life would have been in the hundreds, not tens of thousands,” he says.
But the optimistic researcher also likes to point out demonstrations of the progress in earthquake engineering manifested by the Turkey quake. In a case of dreadful timing, Ford Motor Co. had just completed a new auto plant in Turkey—built directly atop a fault—when the quake struck. Youd points to a photograph of an apparently intact boxlike structure intersected by a ripple in the earth at one corner. “The building performed beautifully,” he exclaims proudly. “It’s leaning a little, part of the foundation is cracked, and they’ll probably have to rebuild the whole thing.” He pauses respectfully, almost as if observing a moment of silence for Ford’s corporate budget. “But if there were any workers in there during the quake, none of them would have been hurt, and that’s what it’s all about for earthquake engineers.”
Tom O’Rourke, a professor of civil engineering at Cornell University and vice president of EERI, appointed Youd to head the mission to Turkey.
“We owe Les a lot because we captured a lot of good information that we wouldn’t have had without his leadership,” says O’Rourke. “He’s also a very talented field geologist, and as a consequence of his powers of observation, he is able to identify various forms of ground deformation. Nobody can do work in the area of liquefaction that doesn’t use some terminology or concepts that he originated.”
Youd likes to say that an earthquake aftermath is his laboratory. And it’s true: you can’t walk into a lab and pour an earthquake into a beaker to start testing it. Geotechnical engineers who study liquefaction have historically been frustrated at having to wait for an earthquake to strike before they test new techniques. Enter Rollins.
The Earthquake Maker
One day over lunch in 1996, Youd and Rollins were lamenting the difficulty of gathering good data from earthquakes. They started brainstorming ways to wire the ground and create their own quakes. First they discussed borrowing huge oil-prospecting trucks and using them to shake the earth in areas prone to liquefaction. But they worried the expensive vehicles might sink and get stuck in the subsequent quicksand. Then one of them proposed using explosives. Thus began the chain of events that would transform Kyle M. Rollins into BYU‘s version of Wyle E. Coyote.
No stranger to inventing unorthodox solutions to geotechnical engineering problems, Rollins had already “broken new ground” in soil compaction research by cranking a 4-ton weight 80 feet in the air and allowing it to plummet onto the same plot of earth seven times. He supervised the technique when it was used to shore up runways at the Provo Airport against future earthquake damage.
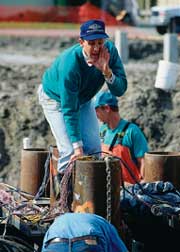
BYU professor Kyle Rollins prepares final details for the first-ever life-sized simulation of liquefaction, a geologic phenomenon that occurs when an earthquake “liquefies” the soil.
But dropping a weight is a little less complicated than setting off enough explosives to create a mini-earthquake. After soliciting sponsorship from the Federal Highway Administration (FHA) and six state Departments of Transportation (DOTs), Rollins and graduate students J. Dusty Lane, A. Tom MacDougall, and Aaron K. McCain threw themselves into months of furious preparation.
In February 1999 Rollins found himself pacing the pit on Treasure Island, watching a demolitions expert pack 16 charges of ammonium nitrate 12 feet under the sandy soil. His hair drenched in the steady drizzle, his coat’s hood waving forgotten in the breeze, Rollins nervously checked and rechecked the hundreds of components of his intricate experiment.
An array of nine steel pillars, or “piles,” each 42 feet long and plastered with 225 sensors, had been driven into the soil just as they would be at the base of a bridge like the one spanning the San Francisco Bay on the horizon. A 15-foot-long hydraulic press, like a giant tire jack on its side, waited to exert horizontal force on the piles to simulate the swaying of a bridge or building during an earthquake.
Six miles of wires from those contraptions snaked their way up the side of the pit and into the camping-trailer-like portable lab. Inside, Rollins silently looked over the shoulders of technicians as they clicked away at laptops, preparing to record signals from buried sensors that would gauge fluctuations in water pressure and the force (or lack thereof) of the soil against the piles.
A gaggle of spectators, consisting mainly of UC Berkeley professors and representatives from the sponsoring state DOTs, huddled impatiently under a stand of pine trees across the pit from the portable lab, trying to escape the rain.
Finally, after 45 minutes of fixing a downed computer program, several months of project delays, and many nights of nagging doubts, Rollins swallowed hard and gave the go-ahead to the demolition expert in the black hard hat.
Before spectators could process the sudden realization that the technician who controlled the explosion was wearing a hard hat and they weren’t, he yelled, “3, 2, 1 . . . fire in the hole!” and let a representative from the FHA turn the crank.
When the demolition man gave the all clear after the blast, Rollins and the other researchers congregated at the edge of the pit, cameras and camcorders in hand. The computers would reveal later if the water pressure had changed enough to create liquefaction, but there was a more obvious way to tell if the experiment was a success.
After a few minutes, Rollins got what he’d been waiting for. Miniature geysers appeared, building cone-shaped mounds of sand around them. Called “sand boils,” the approximately 4-foot-high minivolcanoes are chronic symptoms of liquefaction.
Only when the sand boils appeared did a smile ease its way onto Rollins’ face, and he finally allowed himself to revel in creating the first-ever life-sized simulation of liquefaction. With the hydraulic press pushing back and forth on the piles behind him and the sensors recording how much strength the soil had lost, Rollins exclaimed, “It’s a beautiful test—look at that sand boil!”
Since then, Rollins and his students have been analyzing their data in order to pass along the results to working engineers. “Until now, engineers have had to rely on 30-inch-long models and educated guesses when trying to build earthquake-safe structures in liquefiable soil,” says Rollins, who teamed with UC San Diego’s Scott Ashford on the project. “This test provided the first hard data in the world for designers to base their plans on.”
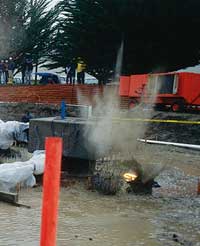
Sending water into the air and shock waves through the ground, 16 explosive charges simulate an earthquake on San Francisco Bay’s Treasure Island. The February 1999 test, conducted by Rollins, yielded the first hard data about how structures behave in liquefiable soil during an earthquake.
So far Rollins has found that liquefied ground offers extremely little resistance to foundations when they move a few inches but more strength than engineers have believed after structures move a few feet. He and Ashford have presented their preliminary findings at seven different conferences, including the 12th World Conference on Earthquake Engineering in Auckland, New Zealand, in February 2000. Their experiment has been met with excitement in the engineering field.
“Currently there is very little design information regarding the lateral response of pile groups in liquefied soils based on full scale testing,” says Jan Six, an engineer with the Oregon Department of Transportation, which co-sponsored the Treasure Island tests. “Design parameters are based on very limited data and largely on engineering judgment. This research should help in refining those judgments and in developing better design parameters.”
The experiment has also yielded other positive results. In an effort to mitigate the effects of liquefaction, Rollins pounded plastic drain hoses about 6 inches in diameter into the ground prior to the blasts. When the “earthquake” occurred, the tubes channeled the surging water into the air, preserving much of the integrity of the soil. California officials say they are going to include such hoses in the ramp to the new San Francisco Bay Bridge they are designing.
Even while analyzing and reporting the results from Treasure Island, Rollins tried another unique method of experimentation. In December, he ordered a visit by the Statnamic, a rocket-powered 39-ton sled the size of a sport utility vehicle. Engineers use it to simulate the immense and sudden force of an earthquake. After sinking another configuration of nine steel piles into the ground near Salt Lake City’s Interstate 15, Rollins aimed the sled at the mock foundation and lit the carbon pellets that fuel it.
In a flash of orange flame and white smoke, the sled slammed into the piles, delivering 800,000 pounds of force in 0.2 seconds—power comparable to the monster strength of a real earthquake. Sensors recorded the reaction of the foundation, giving engineers vital information about how strong their designs must be to survive a seismic event.
Rollins combined the two testing techniques this summer in Charleston, South Carolina, where he has been asked to help design and test a new bridge. Engineers there want his guidance in their effort to simulate liquefaction with explosives, a la Treasure Island, and then use the Statnamic to apply horizontal force while the ground liquefies around the foundations they might use.
“I’ve tried to do work that can be applied,” Rollins says. “I hope that working engineers will be able to take my research and apply it in a way that will have a benefit to people.”
Movers and Shakers
From Les Youd’s discovery of lateral spread to Kyle Rollins’ design parameters for safe foundations, the cumulative impact of the pair of BYU professors is being felt throughout the earthquake engineering field.
Youd, 62, has just finished his second stint as chair of BYU‘s Department of Civil and Environmental Engineering, and he has already served on the board of directors of the EERI, been chair of the Utah Seismic Safety Commission, and won numerous research and teaching awards. He is returning to full-time teaching and research.
Rollins, only 42, won the Huber Prize for young researchers in 1999 from the American Society of Civil Engineers, and he has a new earthquake engineering term of his own to his credit. The acronym TILT is beginning to turn up in the research literature—it stands for Treasure Island Liquefaction Test. The tradition of excellence in liquefaction research at BYU that began with Youd will likely carry on.
“Les is one of the key figures in geotechnical engineering, and Kyle, with his work on Treasure Island, is beginning to make his presence felt. They complement each other well,” says Jim Mitchell, who retired recently after 42 years as a professor of civil engineering at UC Berkeley and Virginia Tech. “They’re a real credit to their profession and their institution.”